Module 2D: Supporting Resources for Implementing Optogenetics in the Lab — Getting Started
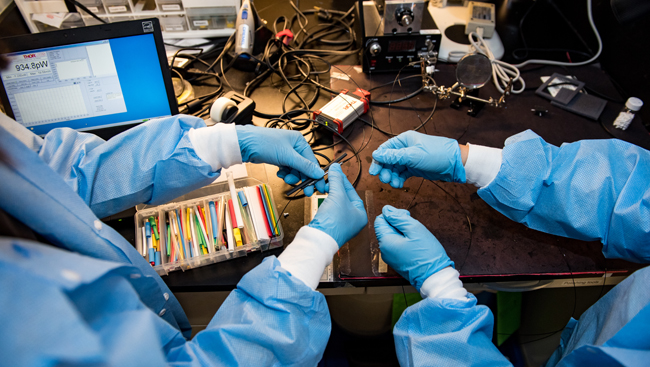
The articles below were selected by Christina Gremel, Vikaas Sohal, and Mario Penzo, faculty from Module 2 of SfN’s Optogenetics Training Series. These resources supplement their presentations, Implementing Optogenetics in the Lab: Why Use Optogenetic Methods, Selection of Opsins, and Delivery of Opsins.
Use these resources to understand when to choose optogenetics versus other approaches, pros and cons of different opsin delivery methods, and available sources of optogenetic tools.
Resources Related to Christina Gremel’s Presentation, Implementing Optogenetics in the Lab: Getting Started
Differential Corticostriatal Plasticity During Fast and Slow Motor Skill Learning in Mice
In this 2011 article, Costa, Cohen, and Nicolelis demonstrate putative single neuron recordings in mice during behavior.
Causal Role for the Subthalamic Nucleus in Interrupting Behavior
Fife et al. demonstrate the use of an inhibitory opsin to test the necessity of a population of cells for a given behavior.
Visualizing Hypothalamic Network Dynamics for Appetitive and Consummatory Behaviors
This 2015 report from Jennings et al. demonstrates the use of multiple methods, including chemogenetic and optogenetic activity tools, to examine the contribution of a specific cell-type to a given behavior.
Distinct Behavioral and Network Correlates of Two Interneuron Types in Prefrontal Cortex
Kvitsiani et al. reveal how optogenetic tagging can be used to examine the neural activity of an isolated cell type during a given behavior.
Lima et al. show how optogenetics can be used to tag neural activity in an identified neuronal population.
In this seminal paper from 1958, Olds reports using intra-cranial self-stimulation to identify a brain area that supports food seeking.
Owen, Berke, and Kreitzer provide a demonstration of combining methods, including cell-type-specific ablation, optogenetics, electrophysiology, imaging, and behavior, to modulate one cell population while recording the activity of a connected cell population.
Whole-Cell Recording of Neuronal Membrane Potential During Behavior
In this 2017 review article, Peterson gives a comprehensive overview of in vivo whole cell recording methods.
Lateral Hypothalamic Circuits for Feeding and Reward
Stuber and Wise review what optogenetics offers in the probing of behavior compared to other techniques.
Resources Related to Vikaas Sohal’s Presentation, Selection of Opsins
Multimodal Fast Optical Interrogation of Neural Circuitry
This seminal 2007 paper from Zhang et al. presents halorhodopsin (NpHR), a complement to channelrhodopsin (ChR2), as a tool capable of generating a chloride flux when activated by light — thereby inducing rapid and reversible hyperpolarization.
Han and Boyden further characterize the halorhodopsin/channelrhodopsin system for multichannel photoinhibition and photostimulation of virally or transgenically targeted neural circuits.
Parvalbumin Neurons and Gamma Rhythms Enhance Cortical Circuit Performance
In this 2009 article, Sohahl, Zhang, et al. use optogenetics to report evidence for the involvement of the specific activation of parvalbumin positive, fast-spiking interneurons in the production of cortical gamma oscillations.
High-Performance Genetically Targetable Optical Neural Silencing by Light-Driven Proton Pumps
Chow et al. describe archaerhodopsin-3 (Arch), a light-driven proton pump that mediates functional neuronal silencing.
Lateral Competition for Cortical Space by Layer-Specific Horizontal Circuits
Adesnick and Scanziani use a combination of optogenetics and electrophysiology to characterize the activity of horizontal projections between vertical domains of the mouse somatosensory cortex.
In this 2010 technical report, Gunaydin et al. describe a novel engineered opsin gene, ChETA, that overcomes many of the technical limitations of channelrhodopsin and allows for sustained spike trains up to at least 200 Hz.
Neocortical Excitation/Inhibition Balance in Information Processing and Social Dysfunction
Yizhar and Fenno, et al. design and use several novel optogenetic tools, including stabilized step function opsins (SFOs), to test the hypothesis that abnormal circuit level physiology (via a cellular excitation/inhibition balance) underlies neuropsychiatric disease-related symptoms.
Mattis et al. present a systematic comparison of microbial opsins — including depolarizing, ultrafast depolarizing, and hyperpolarizing tools — under matched, ex vivo experimental conditions. The authors identify key parameters for the conduct, design, and interpretation of experiments involving optogenetic techniques.
Independent Optical Excitation of Distinct Neural Populations
In this 2014 article, Klapoetke et al. describe two channelrhodopsins, Chronos and Chrimson, that enable two-color activation of independent neural populations without discernable cross-talk.
Projections From Neocortex Mediate Top-Down Control of Memory Retrieval
Rajasethupathy et al. employ a variety of optogenetic approaches to identify a projection from the prefrontal cortex to the hippocampus which, when selectively activated, elicits memory retrieval.
Structural Foundations of Optogenetics: Determinants of Channelrhodopsin Ion Selectivity
Berndt and colleagues identify structural determinants of channelrhodopsin ion selectivity and use their parameters to engineer chloride-conducting channels with higher chloride conductivity and selectivity and test their efficacy in vivo.
Resources Related to Mario Penzo’s Presentation, Delivery of Opsins
Sources of Optogenetic Tools: Viral Vectors
You can purchase a stock viral vector from academic or non-profit viral vector cores. Alternatively, these cores will also develop custom viral vectors from a plasmid that you provide.
- UNC Vector Core at the University of North Carolina at Chapel Hill
- Penn Vector Core at the University of Pennsylvania
- Addgene, the nonprofit plasmid repository
- PVM at IGMM (Platforme de Vectorologie de Montpellier at Institut Génétique Moléculaire Montpellier)
- Private companies can also custom-make viruses from a plasmid that you provide.
Viral Vector Types:
Viral vectors provide a powerful approach for transducing DNA into cells. Many factors must be considered when selecting the appropriate viral vector, including pathogenesis, target cell type, target expression levels, and the size of the genetic cargo.
The table below summarizes the primary factors to consider when making this selection for gene delivery. Table 1 is adapted with permission from AAV, Adenovirus and Lentivirus-Based Gene Delivery Handbook of Vigene Biosciences.
Table 1: Viral Vector Types
|
|||
|
Adeno-associated Virus (AAV) |
Adenovirus
|
Lentivirus
|
Genome |
ssDNA |
dsDNA |
ssRNA (+) |
Coat |
Naked |
Naked |
Enveloped |
Genome size |
5 kb |
38-39 kb |
9 kb |
Infection/tropism |
Dividing and non- dividing cells |
Dividing and non- dividing cells |
Dividing and non- dividing cells |
Host Genome Interaction |
Non-integrating |
Non-integrating |
Integrating |
Transgene expression |
Potentially long-lasting |
Transient |
Long-lasting |
Packaging Capacity |
4.5 kb |
7.5 kb |
6 kb |
Immune Response |
Very Low |
High |
Low |
Relative Viral Titer |
10^7 without concentration |
10^11 without purification |
10^7 without concentration |
Relative Transduction Efficiency |
70 percent |
100 percent |
70 percent |
Relative Foreign Gene Expression |
Medium |
High |
Medium |
More details |
AAV packaging service |
Adenoviral packaging service |
Lentiviral packaging service |
Adeno-Associated Virus Serotypes:
Adeno-associated viruses, or AAVs, are small, single-stranded DNA viruses that are non-pathogenic and can mediate long-term gene expression in vivo. Selecting the right serotype is critical for the success of gene delivery, as one must consider different tissue tropism, anterograde vs. retrograde expression, and other factors.
Table 2 below, adapted with permission from the AAV, Adenovirus and Lentivirus-Based Gene Delivery Handbook of Vigene Biosciences, lists different AAV serotypes often used in neuroscience experiments; commonly used AAVs are in red.
Table 2: Adeno-Associated Virus (AAV) Serotypes
|
|
Serotype |
Notes |
AAV1 |
Some anterograde activity reported (Zingg et al., Neuron, 2017) |
AAV2 |
|
AA5 |
Some retrograde activity reported (Aschauer et al., PLoS One, 2013) |
AAV6 |
|
AAV8 |
|
AAV9 |
Some anterograde activity reported (Zingg et al., Neuron, 2017) |
AAV2-retro |
Exclusive retrograde activity (Tervo et al., Neuron, 2016) |
AAV-PHP.S |
Crosses the blood-brain barrier and selectively transduces the peripheral nervous system. |
AAV-PHP.eB |
Crosses the blood-brain barrier and selectively transduces the central nervous system. |
For more information on viral vectors, please consult:
- AAV guides, such as these from Addgene and Vigen Biosciences
- Retrograde AAV guides, such as from Addgene
- Canine adenovirus type 2( CAV2), an alternative to retrograde AAV, with more information available here
Commonly Used Promoters for Viral Vectors:
Promoters allow for selective expression in viral vectors in different tissues or different brain cells (neurons versus astrocytes) based on gene expression.
The table below, adapted with permission from the AAV, Adenovirus and Lentivirus-Based Gene Delivery Handbook of Vigene Biosciences, lists different promoters that are compatible AAVs and often used in neuroscience experiments.
Table 3: Commonly Used Promoters
|
||
Promoter |
Size |
Description |
CamKIIa |
1.2 kb |
Specific expression in excitatory neurons in the neocortex and hippocampus |
CAG |
944 bp |
Strong promoter, ubiquitous expression in vivo |
EF1A |
1.2 kb |
Ubiquitously expressed; weaker than CMV but better for in vivo |
GFAP |
2.0 kb |
Astrocyte-specific expression |
Synapsin (Syn) |
471 bp |
Specific in neuron |
c-fos |
1.7 kb |
Activity-dependent promoter |
Somatostatin |
1.2 kb |
Expression restricted to some GABAergic neurons |
D2SP |
1.5kb |
Expression restricted to D2R+ neurons |
Mecp2 |
230 bp |
Truncated Mcep2 neuron specific |
Supporting References:
Chan et al., describe a cell type-specific capsid selection method, known as CREATE, was used to produce AAVs that allow gene delivery to the entire central and peripheral nervous systems, with multicolor labeling of single cells.
Non-Invasive, Focused Ultrasound-Facilitated Gene Delivery for Optogenetics
In this 2017 paper, Wang et al. present a noninvasive, ultrasound-based technique to introduce optogenetic channels into the brain by temporarily opening the blood-brain barrier.
Here, Aschauer, Kreuz, and Rumpel provide a detailed and quantitative analysis of the transduction profiles of rAAV vectors based on six of the most commonly used serotypes (AAV1, AAV2, AAV5, AAV6, AAV8, and AAV9) that allows systematic comparison and selection of the optimal vector for a specific application.
A Designer AAV Variant Permits Efficient Retrograde Access to Projection Neurons
Ternvo et al. describe the engineering of a novel AAV vector with potent retrograde functionality (rAAV2-retro) and the resulting retrograde access to projection neurons.
In this 2017 article, Zingg et al. report that adeno-associated virus (AAV1 and AAV9) exhibit anterograde transsynaptic spread properties and allow the tracing and functional manipulation of axonal projections from input-defined neuronal populations.
Nucleus Accumbens D2R Cells Signal Prior Outcomes and Control Risky Decision-Making
Zalocusky et al. report how using a D2 specific promoter enables genetic access to D2R-expressing cells in the rat striatum to exert optogenetic control during decision-making and reveal a causal effect of these cells in driving risk-preference.
Optogenetic Astrocyte Activation Modulates Response Selectivity of Visual Cortex Neurons In Vivo
Perea et al. provide an illustrative example of using astrocyte-specific promoter to drive channelrhodopsin-2 expression. The authors report selective photostimulation of astrocytes with channelrhodopsin-2 in primary visual cortex enhances excitatory and inhibitory synaptic transmission, and influences neuronal integrative features critical for sensory information processing.
A Direct GABAergic Output From the Basal Ganglia to Frontal Cortex
This 2015 paper from Saunders et al. provides an example of using a transgenic mouse line to drive channelrhodopsin-2 expression. The authors use this approach to identify a direct, GABAergic projection from the basal ganglia to frontal regions of the cerebral cortex.
Experience-Dependent Modification of a Central Amygdala Fear Circuit
In this 2013 article, Li, Penzo et al. use a combination of viral vector transduction with a transgenic, Cre-expressing mouse line to express channelrhodopsin-2 or archaerhodopsin and demonstrate robust fear conditioning-induced synaptic plasticity in the central amygdala.
Visit the Community forum for all eight modules to share your insights and best practices, ask questions, and engage with other training series’ participants.
Complete this short survey to provide valuable feedback about Module 2 to SfN and the series faculty.