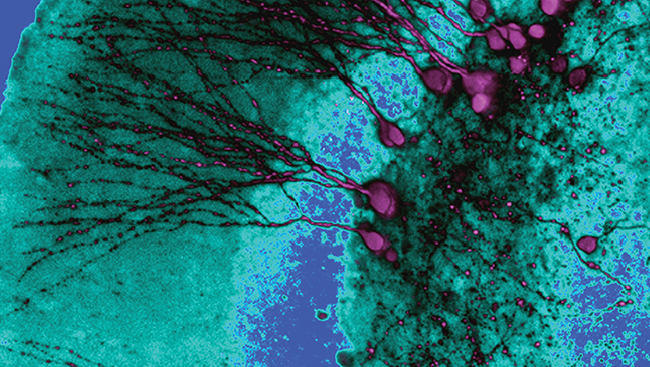
Material below is adapted from the SfN Short Course Mapping Brain Circuitry with a Light Microscope, by Pavel Osten MD, PhD and Troy W. Margrie, PhD. Short Courses are daylong scientific trainings on emerging neuroscience topics and research techniques held the day before SfN’s annual meeting.
Since the days of Camillo Golgi and Santiago Ramón Y Cajal, light microscopes have provided neuroscientists a glimpse into the anatomy of the brain. Advances in the microscopes themselves and methods to stain and trace neurons have revealed various pathways and structures, as well as organization and hierarchies specific to certain brain regions. Three large projects (the Mouse Brain Architecture Project, the Allen Mouse Brain Connectivity Atlas, and the Mouse Connectome Project) are currently attempting to take our knowledge to another level, by mapping all the connections within the brain. Together with new technologies, these projects are helping bring the possibility of a complete map of the mouse brain within reach.
Recent developments of automated light microscopy instruments significantly increase the amount of data collected while reducing the time researchers spend collecting it. One automated approach combines two-photon microscopy with tissue sectioning, such as in serial two-photon tomography. A second approach is light-sheet fluorescence microscopy, which uses chemicals to render mouse brains transparent. CLARITY, a new twist on this method, avoids earlier problems with the clearing chemicals dimming fluorescent signals.
To identify the connections, anterograde tracers, retrograde tracers and viruses are used. Anterograde tracers label projections as they are transported from the cell body towards the axon terminal, while retrograde tracers label as they move backwards, from the terminal towards the cell body. To visualize specific synaptic contacts, viruses that can cross one or more synapses can be used. Viruses can also be targeted to particular cells by combining them with other viruses expressing specific receptors or with Cre-recombinase transgenic mice, enabling mapping within selective brain regions or cell types. Each of the three projects uses a different combination of these strategies to complement each other and allow for cross-validation of their results.
Challenges associated with charting all the connections include the sheer size of the datasets (several terabytes per brain), registering each brain onto a reference atlas, and ensuring standardized analytical methods are used across the different research groups. Additionally, these tracing methods do not allow the function of circuits to be probed, only the structure. But, by combining methods used for mapping connections with single-cell electrophysiology or calcium imaging, questions about functions of certain cells within a network can be addressed. Specific cell types can then be targeted with optogenetics, allowing causality to be tested. Together these techniques will enable the creation of a complete structure-function map for different behaviors.
Like the connectome of the roundworm C. elegans, a complete mouse brain connectome will reveal the entire brain’s circuitry and provide a starting point for further research. It will allow the various functions of different circuits to be probed, telling us which neurons are responsible for certain behaviors, and help us understand diseases caused by disruptions to these circuits. Light microscopy instruments have come a long way from the days of Golgi and Ramón Y Cajal, and we currently stand on the cusp of a detailed understanding of all the connections, both local and long-range, within the mouse brain.