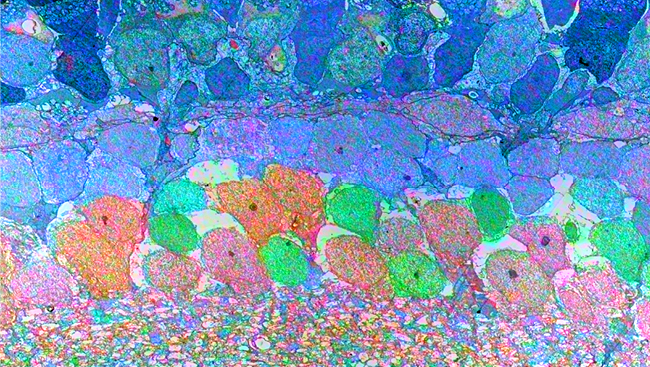
Material below summarizes the article Organization of the Mammalian Locomotor CPG: Review of Computational Model and Circuit Architectures Based on Genetically Identified Spinal Interneurons, published on September 8, 2015, in eNeuro and authored by Ilya A. Rybak, Kimberly J. Dougherty, and Natalia A. Shevtsova.
Locomotion represents motor behaviors that allow animals to move in physical environment. In legged animals, including mammals, locomotion results from coordinated rhythmic movements of limbs interacting with the ground. As in other rhythmic behaviors, such as breathing or scratching, locomotion is controlled by central neural circuits referred to as central pattern generators (CPGs). The CPGs are able to autonomously generate basic rhythmic activity without rhythmic inputs from the higher brain areas and without afferent feedback.
The CPGs that generate the basic rhythmic locomotor activity in all vertebrates, including mammals, are located in the spinal cord. It is considered that there is at least a single CPG controlling each limb, since rats, cats, and humans are able walk on treadmills with split belts moving with different speeds. The locomotor pattern generated by a single CPG consists of two major phases, flexion and extension, which correspond to the swing (when limb is moving to a new position) and stance (when limb is standing on the ground) phases, respectively, in walking. It has been proposed that this flexor-extensor pattern is generated by reciprocally interacting flexor and extensor half-centers in each CPG. Interactions between circuits (and CPGs) controlling left and right limbs are mediated by commissural interneurons (CINs), whose axons cross the spinal cord midline and affect circuits on the contra-lateral side of the cord.
The generation of locomotor rhythm and pattern can be studied in vitro, using isolated spinal cord preparations from neonatal rodents. The locomotor activity in these preparations is usually evoked by a combination of physiological active drugs (typically, NMDA and serotonin) added to the solution.
Having a complete picture of the circuitry of the locomotor CPG is important for understanding the way in which movements are generated. In addition, this knowledge can provide insights into treatments following spinal cord injury and other motor disorders. In contrast to many other neural networks in the brain, whose architecture, neuron types, and connectivity have been mainly or partly identified, the spinal cord circuitry does not exhibit a clear spatial organization, which makes it difficult to identify network elements with specific functions using traditional electrophysiological methods. Significant progress in this area has been achieved due to the classification of spinal interneurons based on their progenitor domains and the transcription factors they express during early developmental stages. These methods allowed researchers to identify several groups of spinal interneurons (V0-V3 types) involved in the CPG operation and commissural interactions between left and right circuits.
Due to the genetic amenability of the mouse, the in vitro neonatal mouse spinal cord preparation has been a leading system for the study of genetically identified neural populations. Over the past decade, transgenic mice have been used to genetically ablate or silence various populations of interneurons. Isolated spinal cords from these transgenic mice have been used to evaluate the locomotor consequence of ablating/silencing each of the identified population. Although we now know, for example, that V0 and V2a interneurons are involved in left-right coordination at different speeds and V1 and V2b interneurons are essential for flexor-extensor alternation, their circuit connectivity cannot be extracted from these experiments alone.
The interplay between computational modeling and experimental studies has been valuable in advancing our understandings of different vertebrate CPGs. Using this strategy, experimental data from multiple labs can be compiled and incorporated to create, validate, and/or refine computation models. These models can suggest plausible network architectures that are consistent with multiple experimental findings and generate specific hypotheses that can be tested experimentally.
In this paper, we review the results of recent experimental and modeling studies and present common connectivity schematics of the spinal network, which include most of genetically identified neuron populations within the spinal cord. These populations are briefly described below.
First, there are three distinct types of populations of ipsilaterally-projecting excitatory interneurons. One type represents the populations of rhythm-generating interneurons (Shox2, non-V2a, and currently unidentified neurons) that are subdivided into flexor and extensor populations (CPG half-centers). Next, there are populations of excitatory neurons (V2a type I or Shox2- V2a) that receive inputs from the rhythm-generating flexor half-centers and provide excitation to some (V0V) CIN populations. These neurons are essential for left-right alternation of neuron activity in the cord at higher locomotor frequencies. Last, there are populations of excitatory interneurons (Shox2+ V2a or V2a type II) that mediate CPG inputs to the ipsilateral flexor and extensor motoneurons controlling corresponding limb muscles.
Second, there are two ipsilaterally projecting populations of inhibitory neurons, V1 and V2b, that are involved in inhibitory flexor-extensor interactions within each CPG. Current data suggests an asymmetric organization of these interactions. In the proposed model, only the flexor half-center in each CPG intrinsically generates rhythmic activity, whereas the extensor half-center operates in a sustained activity regime and exhibits rhythmic activity only because of the rhythmic inhibition from the corresponding flexor half-center. According to the suggested schematic, the V2b populations mediate reciprocal inhibitory interactions between the flexor and extensor half-centers in each CPG. The V1 populations are involved in speeding up the locomotor activity and are also involved in flexor-extensor interactions on ipsilateral side of the cord, receiving inputs from the contralateral side.
Finally, three distinct CIN populations control left-right interactions in the cord. The populations of V0D inhibitory CINs provide direct inhibition of the contralateral rhythm generating (flexor) half-centers and secure left-right alternation of activity in the cord at lower locomotor speed. The populations of V0V excitatory CINs also inhibit the contralateral rhythm generator neurons (via interposed inhibitory interneurons), but secure left-right alternation at higher locomotor speeds. In contrast to both V0 CIN types promoting left-right alternation, the excitatory V3 CINs promote synchronization of activity in the left and right sides of the cord by direct excitation of the contralateral rhythm generating (flexor) half-centers. Therefore, elimination of both V0 CIN types causes switching from a normal left-right alternating activity to a left-right synchronous “hopping” pattern. Moreover, selective ablation of the inhibitory V0D CINs results in the lack of left-right alternation at low locomotor frequencies but retained alternation at high frequencies, whereas selective ablation of the excitatory V0V CINs or V2a neurons maintains the left–right alternation at low frequencies and switches to a hopping pattern at high frequencies.
The network organization in the spinal cord proposed and analyzed in this study provides explanations for experimental data obtained in different laboratories under different experimental conditions and suggests the exact functional roles and connectivity patterns of several genetically identified types of spinal interneurons. The modeling predictions include:
- Flexor–extensor asymmetry in the organization of rhythm-generating locomotor network.
- Organization and frequency-dependent interactions between commissural pathways supporting left–right alternation (V0D, V2a/V0V) and commissural pathways promoting left-right synchronization (V3), all of which are potentially involved in locomotor gait control.
- Organization of circuits of ipsilaterally projecting V1 and V2b neurons providing flexor–extensor alteration and control of locomotor speed. These predictions provide important insights into the organization of spinal locomotor CPGs and neural control of locomotion, and await experimental testing and validation.
Visit eNeuro to read the original article and explore other content. Read other summaries of JNeurosci and eNeuro papers in the Neuronline collection SfN Journals: Research Article Summaries.
Organization of the Mammalian Locomotor CPG: Review of Computational Model and Circuit Architectures Based on Genetically Identified Spinal Interneurons. Ilya A. Rybak, Kimberly J. Dougherty, Natalia A. Shevtsova. eNeuro Sep 2015, 2 (5) DOI: 10.1523/ENEURO.0069-15.2015