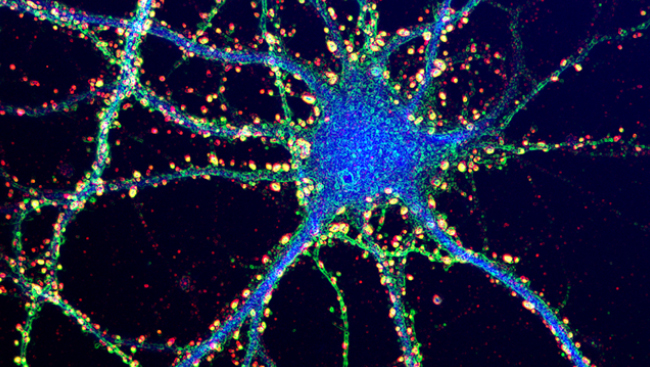
Material below is adapted from the SfN Short Course Advances in Multi-Neuronal Monitoring of Brain Activity, by Douglas S. Kim, PhD, Vivek Jayaraman, PhD, Loren L. Looger, PhD, and Karel Svoboda, PhD. Short Courses are daylong scientific trainings on emerging neuroscience topics and research techniques held the day before SfN’s annual meeting.
As neuroscientists try to understand the dynamics of both large networks of neurons and single cells, new methods to monitor neuronal activity are being developed. One way to study synaptic and action potential activity is to measure intracellular calcium. Within dendritic spines, calcium transients correlate with synaptic activity, and in cell bodies, calcium signals accurately reflect action potentials (spikes).
Early techniques to visualize calcium involved synthetic dyes that emit light in proportion to the amount of calcium present. But these dyes are not specific in that astrocytes and other cells are also labeled. In addition, synthetic dyes cannot be used for repeated, long-term imaging. Newer sensors such as genetically encoded calcium indicators (or GECIs) are delivered by viruses or bred into transgenic animals, allowing expression that can be both long term and targeted to specific cell types. They can be used both in cell cultures and living animals and optimized for specific research questions.
One such GECI is GCaMP, a fusion protein linking green fluorescent protein to calmodulin and the M13 domain of myosin light chain kinase. When calcium binds calmodulin, calmodulin binds M13. These interactions change the structure of the protein complex, allowing it to glow green where the brightness is proportional to the increase in calcium.
At first, GECIs had poor temporal resolution, but sensitivity has improved dramatically. For example, when mice were shown different stimuli, one GCaMP variant was able to detect single spikes in visual cortical neurons. Furthermore, the same neurons could be imaged for months.
To optimize imaging for different cell types, future manipulations will also tweak binding affinity. In cells with high frequency activity, the signals get easily saturated; a lower binding affinity would improve detection. Alternatively, a higher affinity would be helpful in cells in which calcium is quickly buffered, like parvalbumin-expressing interneurons.
In addition to green fluorescent calcium sensors, red fluorescent sensors have also been created. Red light can penetrate farther into tissue, enabling deeper and less invasive imaging than green. Red is also more compatible with common optogenetic methods that use blue light, like channelrhodopsin-2-based experiments. Newer red GECIs are beginning to approach the sensitivity of green GECIs, although bleaching remains a problem.
As GECIs continue to improve, future possibilities for the technology are exciting. For example, combining red and green sensors will allow imaging of multiple cell types simultaneously. GECIs provide researchers a way to witness the dynamics of cell communication across networks and within sub-compartments of an individual cell in a whole new light.