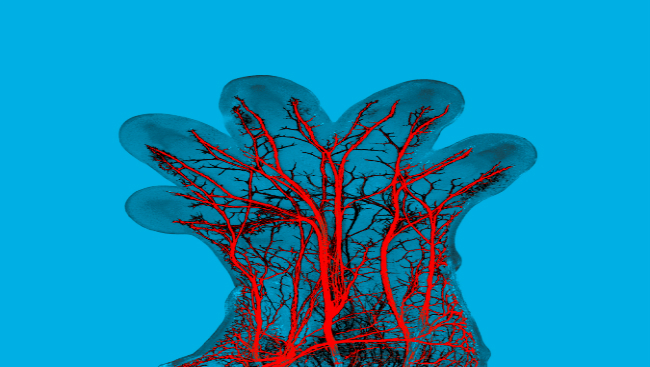
Material below summarizes the article Activity Patterns in the Neuropil of Striatal Cholinergic Interneurons in Freely Moving Mice Represent Their Collective Spiking Dynamics, published on January 4, 2019, in eNeuro and authored by Rotem Rehani, Yara Atamna, Lior Tiroshi, Wei-Hua Chiu, José de Jesús Aceves Buendía, Gabriela J. Martins, Gilad A. Jacobson, and Joshua A. Goldberg.
Live imaging of neuronal populations often reveals a background signal that engulfs the signal from individual neurons. Typically, this background signal is dismissed as uninformative or as an epiphenomenon. We imaged in freely moving mice acetylcholine-releasing (cholinergic) interneurons in the striatum that play a critical role in basal ganglia function and dysfunction in movement disorders. Importantly, these interneurons give rise to a profusely dense neuropil of fine neuronal processes that fill the striatum. Under these circumstances, our analysis revealed the background signal arising from the neuropil represents a “mean-field” readout of the collective recurrent activity of cholinergic interneurons. Thus, the neuropil signal functions as a physiological readout of the network state.
For over half a century, clinicians and scientists have known a disruption of the so-called balance between acetylcholine and dopamine released in the region of the brain called the striatum is a central pathological correlate of various movement disorders such as Parkinson’s disease and Huntington’s disease. This imbalance was deduced from biochemical and histological studies of the striatum. However, evidence for such an imbalance in the physiological activity of brain circuits has been lacking.
Only recently have imaging and molecular techniques enabled us to peer directly at the activity of dopamine and acetylcholine circuits in freely moving mice. We can now target specific neuronal types, such as cholinergic interneurons, with genetically encoded fluorescent markers and visualize their activity with tiny and extremely light fluorescent microendoscopes placed on the heads of the mice. We hoped with this technology, we could monitor the activity of cholinergic interneurons and begin to understand how acetylcholine is released in the striatum of freely moving mice.
While we observed signals from individual neurons, what was striking about our striatal imaging in the freely moving mice was the background neuropil signal that surrounds them. It appeared to “light up” in bouts of bright fluorescence that were often far brighter than the signals from the individual neurons. Moreover, this background signal was highly synchronous and correlated across large regions of the striatal neuropil. However, the oddest result by far was that the neuropil signal — while clearly associated with the signals from individual cell bodies — both preceded those signals and decayed more quickly than they did.
What could explain the faster kinetics of the neuropil signal and why it preceded the signals from individual neurons? Moreover, what is the meaning of the synchronous neuropil signal? One possibility is that the background signal represents synaptic input to cholinergic interneurons, which precedes their response. The fact that the background signal is spatially synchronous might mean cholinergic interneurons are engaged synchronously by bouts of common input. In this case, the neuropil signal could be considered a feed-forward signal. Alternatively, the background signal could represent the sum-total of action potentials emitted by a network of cholinergic interneurons. These action potentials presumably spread throughout the neuropil. In this case, the neuropil signal should be considered a feedback or recurrent cholinergic network signal.
By combining advanced imaging and optogenetic techniques we were able to show that although the neuropil signal precedes the signals from individual neurons, it does not represent the input. Rather, it represents a population average of the concurrent activation of many cholinergic interneurons, most of which have cell bodies located outside of the microendoscope’s field of view (e.g., in deeper regions of the striatum). Their neural activity can be observed in the field of view, however, because when action potentials are triggered near their cell bodies, they travel along the axon as well as along dendrites, in a process called back-propagation. The process is so named because the direction goes seemingly “against” the normal flow of information in the neuron, which is supposed to go from dendrites to axon, not vice versa.
Because the dendritic and axonal arbors of cholinergic interneurons that constitute the cholinergic neuropil are exceptionally dense and volume-filling, action potentials from throughout the striatum contribute to the background signal observed in the field of view. The faster kinetics of the neuropil signal are due to neuronal biophysics dictating that signals rise and decay faster in smaller diameter neuronal processes.
If the neuropil signal represents an average population activity, wouldn’t one expect to find the cell body signals precede the average signal in half of the cases? The answer is no. The neuropil signal represents a process of neuronal recruitment, so it is unlikely the neurons in the field of view are among the first recruited. Furthermore, given we imaged superficial layers of the striatum, and the recruitment of cholinergic interneurons most likely originates in the deeper regions of the striatum, superficial interneurons are expected to be recruited only later.
The “mean-field” nature of the neuropil signal is reminiscent of other well-known physiological readouts of population activity, such as the local field potential (LFP), which is also famously synchronous across large distances in the brain. One of the exciting dynamical features of LFP signals is that it has been shown to give rise to traveling waves of activation. We are currently studying the neuropil signal to see whether it, too, reveals such organized spatiotemporal structures in the activation of cholinergic interneurons, particularly in light of our hypothesis that the recruitment of cholinergic interneurons begins in deeper regions of the striatum and spreads from there.
Having revealed the source of the cholinergic neuropil signal, the question still remains: How do we know the neuropil signal is anything more than an epiphenomenon? Future studies will determine how the cholinergic neuropil signal corresponds in a meaningful way with innate or learned, motor or associative mouse behaviors. Moreover, such a robust readout of striatal cholinergic activity (possibly matched with some comparable robust readout of striatal dopaminergic activity) could perhaps one day serve as a biomarker for quantifying the famous dopamine acetylcholine imbalance in movement disorders.
Visit eNeuro to read the original article and explore other content. Read other summaries of JNeurosci and eNeuro papers in the Neuronline collection SfN Journals: Research Article Summaries.
Activity Patterns in the Neuropil of Striatal Cholinergic Interneurons in Freely Moving Mice Represent Their Collective Spiking Dynamics. Rotem Rehani, Yara Atamna, Lior Tiroshi, Wei-Hua Chiu, José de Jesús Aceves Buendía, Gabriela J. Martins, Gilad A. Jacobson, and Joshua A. Goldberg. eNeuro Jan 2019, 6 (1) ENEURO.0351-18.2018; DOI: https://doi.org/10.1523/ENEURO.0351-18.2018